
Towards Achieving Net-zero Emissions from the Co-combustion of Natural Gas and Biomass: A Commercial Pathway for Evaluating the Performance of a Novel Solvent Blend
The pressing need to reduce greenhouse gas emissions, particularly CO2, necessitates a clean-up of the energy sector. Coal, traditionally valued for its abundance, low cost, and reliability, unfortunately emits significant amounts of CO2 contributing to air pollution, acid rain, and climate change issues.....
Read More
Storage and Utilization of CO2 in Ready-mix Concrete Using CO2-loaded Aqueous Inorganic Solvent
Recently, there has been significant attention focused on utilizing captured CO2 to produce valuable materials. An example is sequestering CO2 through mineral carbonation and converting it into a commercially valuable product (Jang et al., 2016. Constr Build Mater, vol. 127, pp. 762–773, Elsevier Ltd).....
Read More
Using Catboost-SMOTE Machine Learning to Discover Brand New Amines which can be Synthesized and Tested for their CO2 Capture Performance
This post highlights a powerful statistical technique known as Synthetic Minority Over-sampling Technique (SMOTE) that was also used in combination with Categorical Boosting (Catboost) technique in our recently published work on development of a predictive degradation model of amine used in CO2 capture process. Theoretically, SMOTE is...
Read More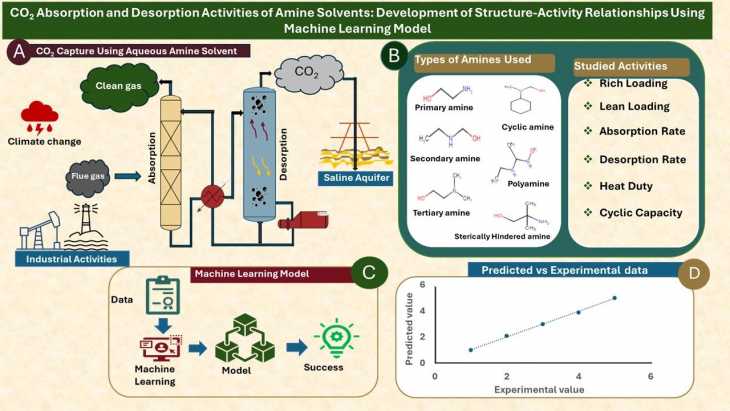
CO2 Absorption and Desorption Activities of Amine Solvents: Modeling of Structure-Activity Relationship Using Machine Learning
Selecting amine solvents for CO2 post-combustion capture application requires an experimental screening of the solvents to determine their performance...
Read More
Sample Microgrid Post #2
Lorem ipsum dolor sit amet, consectetur adipiscing elit. Sed malesuada ligula ac augue mattis cursus. Morbi congue accumsan dolor, non ornare neque maximus at. Mauris eu orci sed risus venenatis ultricies. Suspendisse at leo molestie, facilisis sapien in, luctus ligula.
Read More
Performance Evaluation of a New Novel Amine Bi-Blend Solvent in a Catalyst Aided CO2 Capture Process in a Full Cycle Mini Pilot Plant
The amine-based post-combustion capture method utilizes reactive solvents, amines, to absorb CO2 from industrial flue gas. Despite its proven success, this method faces a significant challenge due to its high energy requirements. Efforts have been made to enhance the process’s efficiency, particularly in reducing its energy demand. One such endeavor involves the development of solvents […]
Read More
Pretreatment by Simultaneous Removal of SO2 and NO2 from Flue Gas
The global concern over climate change has led to the implementation of various measures to reduce greenhouse gas emissions, including carbon capture and storage (CCS) technology.
Read More
AMP and MEA Carbamate Stability Comparison
In this post, we explain why carbamate of sterically hindered AMP is unstable relative to that of MEA.
Read More
Improvement of Biobased Catalyst for Biodiesel Production from Waste Cooking Oil
In order to attain net zero carbon emissions by 2050 and meet a 45% of biofuel production from waste resources target by 2030, there is the need to explore catalysts for increasing biofuel yield. This work will explore the use of environmentally benign materials for biodiesel production.
Read More
Perception in Neural Networks
Neural network is a type of machine learning algorithm that is inspired by the structure and function of the human brain.
Read More